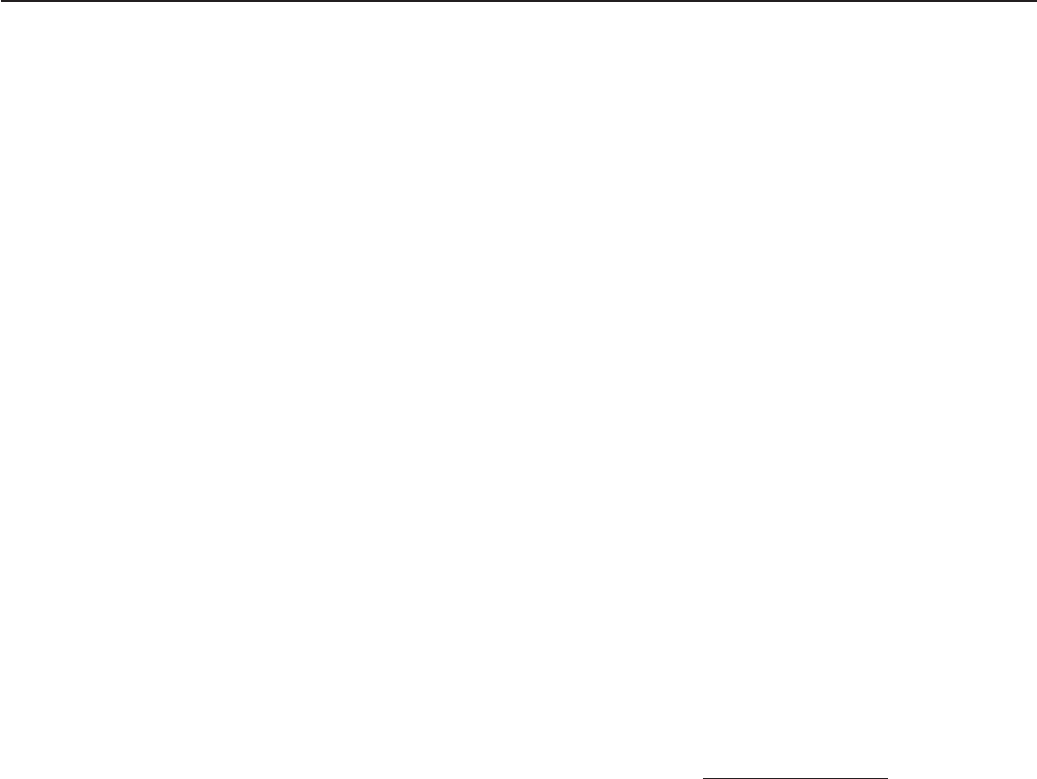
Owejan – General Motors
V.F Fuel Cells / Transport Studies
832
DOE Hydrogen and Fuel Cells Program
FY 2011 Annual Progress Report
FY 2011 Publications/Presentations
1.J.Gagliardo,J.Fagley,D.Fultz,J.Owejan,“Inuence
of Through-Plane Thermal Profile on Water Accumulation
inProtonExchangeMembraneFuelCells”Meetingofthe
Electochemical Society, Las Vegas, NV. October 2010.
2. Jacob M LaManna, Fengyuan Zhang, Subhadeep
Chakraborty, and Matthew M. Mench, Quantification of
Through-Plane Liquid Water Gradients and Transport in
PEFCswithHighResolutionNeutronImaging.Acceptedfor
Presentation, 2011 ECS Meeting, Montreal Canada.
3.
ZijieLu,CodyRath,GuangshengZhang,
Satish G. Kandlikar. Water management studies in PEM fuel
cells,partIV:Effectsofchannelsurfacewettability,geometry
and orientation on the two-phase flow in parallel gas channels,
InternationalJournalofHydrogenEnergy(2011).
4.Kongkanand,A.,“InterfacialWaterTransportMeasurements
inNaonThinFilmsUsingaQuartz-CrystalMicrobalance,”
J. Phys. Chem. C, 115, pp. 11318-11325, (2011).
5. Sergi, J.M., Kandlikar, S.G., “Quantification and
Characterization of Water Coverage in PEMFC Gas Channels
UsingSimultaneousAnodeandCathodeVisualizationand
ImageProcessing,”InternationalJournalofHydrogenEnergy,
Accepted Manuscript, 2011.
6.
J.Owejan,DOEAnnualMeritReview,ArlingtonVA,May
12, 2011.
References
1.Gu,W.,Baker,D.R.,Liu,Y.,Gasteiger,H.A., “Proton
exchangemembranefuelcell(PEMFC)down-the-channel
performancemodel,”Handbook of Fuel Cells - Volume 5, Prof.
Dr. W. Vielstich et al. (Eds.), John Wiley & Sons Ltd., (2009).
2. Owejan, J.P., Gagliardo, J.J., Sergi, J.M., Kandlikar, S.G.,
Trabold, T.A., “Water management studies in PEM fuel
cells,PartI:Fuelcelldesignandinsituwaterdistributions,”
InternationalJournalofHydrogenEnergy,34(8),pp.3436-
3444, (2009).
3.
Kongkanand,A.,“InterfacialWaterTransportMeasurements
inNaonThinFilmsUsingaQuartz-CrystalMicrobalance,”
J. Phys. Chem. C, 115, pp. 11318-11325, (2011).
4.Nolte,A.J.;Treat,N.D.;Cohen,R.E.;Rubner,M.F.
Macromolecules, 41, 5793–5798, (2008).
5.
Miller,K.E.;Krueger,R.H.;Torkelson,J.M.,J.Polym.Sci.:
Polym. Phys, 33, 2343-2349, (1995).
6. Sergi, J.M., Kandlikar, S.G., “Quantification and
Characterization of Water Coverage in PEMFC Gas Channels
UsingSimultaneousAnodeandCathodeVisualizationand
ImageProcessing,”InternationalJournalofHydrogenEnergy,
Accepted Manuscript, 2011.
To optimize the channel design for better water
management, the effects of channel surface wettability,
cross-sectional geometry and orientation on the two-phase
owinparallelgaschannelswereinvestigated.Itwasfound
that hydrophilic channels are advantageous over uncoated
or slightly hydrophobic channels in terms of uniform water
and gas flow distribution and promoting film flow. Stamped
metal geometry channels favor film flow when compared
to a rectangular geometry. Vertical channel orientation is
advantageous over horizontal orientation because it is less
prone to slug flow, and facilitates more uniform liquid water
distribution and stable operation.
Conclusions and Future Directions
A well organized characterization, modeling and
validation framework was developed early in this project.
Therstphaseofexecutionwaslargelyfocusedon
experimentaldevelopmentwithkeyaccomplishments
outlined as follows:
Project is standardized by materials and operating space:•
Baseline and auto-competitive material sets chosen
–
based on parametric variations that consider
degradation and cost vs. performance trade-offs.
Key relationships required for a wet 1+1D model and •
characterization methods are defined:
Subjectmatterexpertsaredevelopingandexecuting –
characterization methods to generate physical
understanding of fundamental processes.
Component models describing processes are being
–
generated and will be used to output bulk and
interfacial transport resistances.
Modeling framework for 1+1D model is defined.
–
Down-the-channel baseline material validation data set •
complete:
Additionalrepeatexperimentsbeingexecutedto –
define uncertainty.
Database on the website for dissemination of data and •
modeling:
Visit www.pemfcdata.org (development will
–
continue throughout the project).
Thenextphaseofthisprojectisfocusedonrening
the component and down-the-channel models in parallel
while repeating characterization and validation work for the
next-generationmaterialset.Thisworkwillsupportthekey
deliverablefornextyear:avalidated1+1Dmodelcapableof
predicting performance and water balance under saturated
conditions with baseline material parameters.