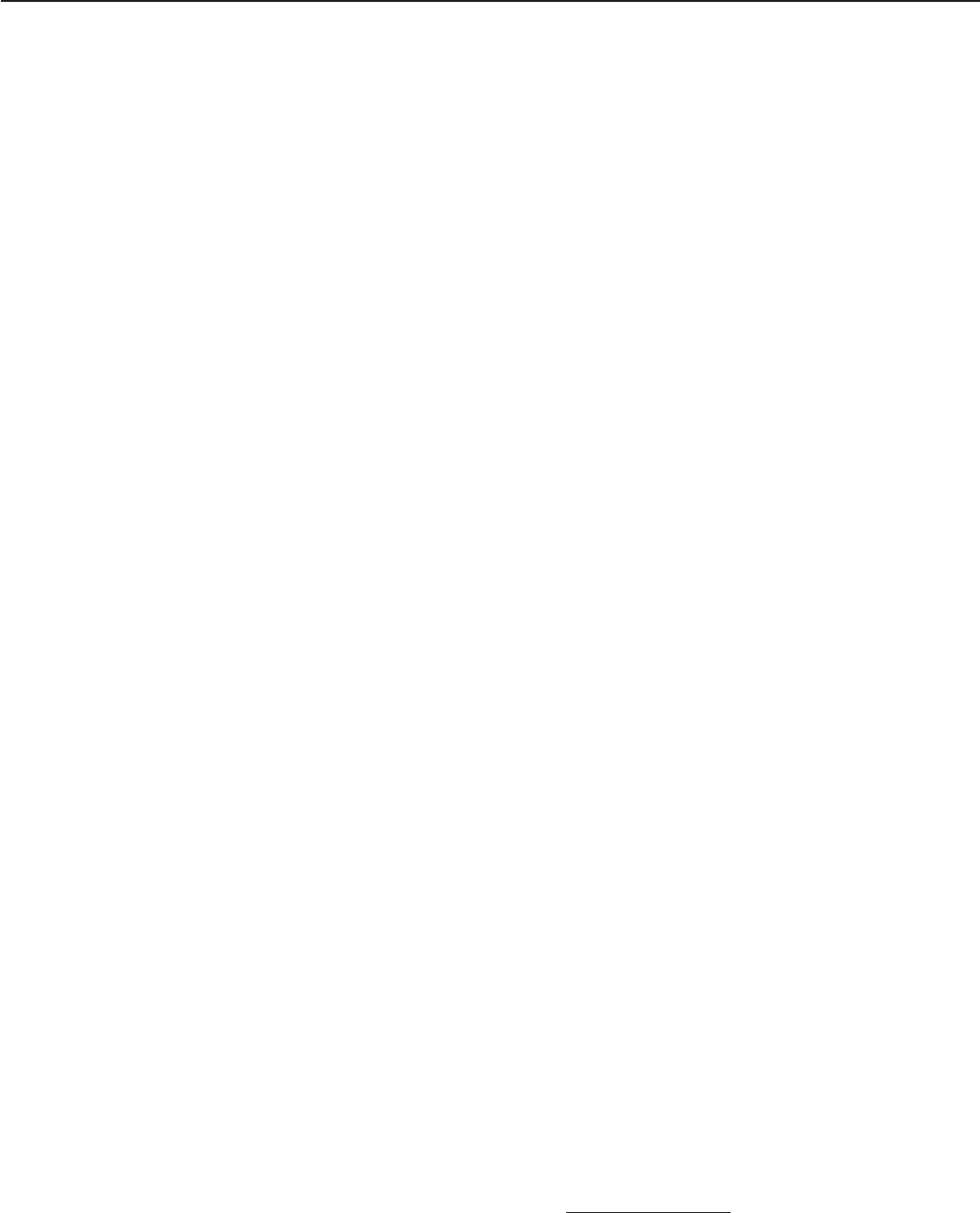
Gu – General Motors
V.G Fuel Cells / Transport Studies
V–156
DOE Hydrogen and Fuel Cells Program
FY 2014 Annual Progress Report
layer of PEM fuel cells,” International Journal of Heat and Mass
Transfer, 71(10), pp. 585-592 (2014).
9. Kandlikar, S.G., E.J.See, M. Koz, P. Gopalan, R. Banerjee, “Two-
PhaseFlowinGDLandReactantChannelsofaProtonExchange
Membrane Fuel Cell,” International Journal of Hydrogen Energy, 39
(12), pp. 6620-6636 (2014).
10. Banerjee, R., and S.G. Kandlikar, “LiquidWaterQuantication
intheCathodeSideGasChannelsofaProtonExchangeMembrane
Fuel Cell Through Two-Phase Flow Visualization,” Journal of
Power Sources, 247, pp. 9-19 (2014).
11. Gopalan, P., and S.G. Kandlikar, “Modeling Dynamic
Interaction Between an Emerging Water Droplet and the Sidewall of
a Trapezoidal Channel,” Colloids and Surfaces A: Physicochemical
and Engineering Aspects, 441, pp. 267-274 (2014).
12. Gopalan, P., and S.G. Kandlikar, “Effect of Channel Materials
and Trapezoidal Corner Angles on Emerging Droplet Behavior in
ProtonExchangeMembraneFuelCellGasChannels,” Journal of
Power Sources, 248, pp. 230-238 (2014).
13. Banerjee, R., E.J. See, and S.G. Kandlikar, “Pressure Drop
and Voltage Response of PEMFC Operation under Transient
Temperature and Loading Conditions,” ECS Transactions, 58(1), pp.
1601-1611 (2013).
14. See, E.J., and S.G. Kandlikar, “Effect of GDL Material on
Thermal Gradients along the Reactant Flow Channels in PEMFCs,”
ECS Transactions, 58(1), pp. 867-880 (2013).
15. Xu, G., LaManna, J., and Mench, M.M., “Measurement of
Thermal Conductivity of Partially Saturated Diffusion Media under
Compression,” 2014 ECS Spring meeting, Toronto, Canada, May
12–16, 2014.
16. LaManna, Jacob M., Matthew M. Mench , “ Flow Field and
DiffusionMediaTortuosityInuencesonPEFCWaterBalance,”
Accepted for invited oral presentation. 2014 ECS and SMEQ Joint
InternationalMeeting,Cancun,Mexico,October5–10,2014.
17. LaManna,Jacob M., Daniel S. Hussey, David L. Jacobson,
Matthew M. Mench, “Role of Land Drainage on Water Storage in
PolymerElectrolyteFuelCellsUsingHighResolutionNeutron
Imaging,” Accepted for poster presentation.10
th
World Conference
on Neutron Radiography. Grindelwald, Switzerland, October 5–10,
2014.
18. Fenton, D.J., J.J. Gagliardo and T.A. Trabold, “Analysis of water
morphology in the active area and channel-to-manifold transitions
of a PEM fuelcell,” submitted for publication in Proceedings of the
ASME 8
th
International Conference on Energy Sustainability &
12
th
International Fuel Cell Science, Engineering and Technology
Conference, Paper ESFuelCell2014-6565, Boston, MA, June 30 –
July 2, 2014.
REFERENCES
1.Gu,W.,Baker,D.R.,Liu,Y.,Gasteiger,H.A.,“Protonexchange
membrane fuel cell (PEMFC) down-the-channel performance
model,” Handbook of Fuel Cells - Volume 5, Prof. Dr. W. Vielstich
et al. (Eds.), John Wiley & Sons Ltd. (2009).
2. Owejan, J.P., 2011 Annual Progress Report for the DOE
Hydrogen and Fuel Cells Program (2011).
Coninuedstudiesonthinionomerlmsandionomer-•
substrate interactions.
Neutron imaging data on liquid water saturation within •
GDLfortheeffectsofGDLtype,aging,andoweld
shows the impact of GDL surface properties and heat
transfer.
Validatedoweldpressuredropmodelincludesthe•
effects of water droplet-channel corner interaction, liquid
waterowpattern,andlocaloperatingcondition.
Signicantpressuredropoccursinthenon-active,•
channel-to-manifold region due to liquid water
accumulation therein; peak active area water volume
existslikelyduetogasmomentumandhydraulicforce
balance.
Down-the-channel 1+1D model improved with new •
relationships integrated, and the opposite trend in down-
the-channel current distribution associated with auto-
competitive material set successfully captured.
A parametric study performed for cell component •
optimization, and optimal material properties
recommended for better cell performance.
TheprojectendedinMay2014.Analreportisforthcoming.
FY 2014 PUBLICATIONS/PRESENTATIONS
1. Petrina, S.A. “Water Sorption, Viscoelastic, and Optical
Properties of Thin NAFION
®
Films.” Ph.D. Dissertation,
PennsylvaniaStateUniversity,2013.
2. Kusoglu, A., D. Kushner, D.K. Paul, K. Karan, M.A. Hickner,
A.Z.Weber,“ImpactofSubstrateandProcessingonConnement
ofNaonThinFilms,”Advanced Functional Materials 2014,
DOI: 10.1002/adfm.201304311.
3. Zimudzi, T.J., M.A. Hickner, “FTIR Analysis of Alignment in
NAFION® Thin Films at SiO
2
and Au Interfaces,” submitted to
J. Phys. Chem. B (2014).
4. Kushner, D.I., M.A. Hickner, “FTIR Analysis of Alignment in
NAFION® Thin Films at SiO
2
and Au Interfaces,” submitted to
Langmuir (2014).
5.LaManna,J.M.,J.M.Bothe,F.Y.Zhang,andM.M.Mench,
“Measurement of Capillary Pressure in Fuel Cell Diffusion Media,
Micro-Porous Layers, Catalyst Layers, and Interfaces,” submitted to
J. Power Sources (2014).
6. LaManna, J.M., Chakraborty, S., Gagliardo, J.J., and
Mench, M.M., “Isolation of transport mechanisms in PEFCs
using high resolution neutron imaging,” International Journal of
Hydrogen Energy, 39(7), pp. 3387-3396 (2014).
7. Xu, G., LaManna, J.M., Clement, J.T., and Mench, M.M., “Direct
measurement of through-plane thermal conductivity of partially
saturated fuel cell diffusion media,” Journal of Power Sources, 256,
pp. 212-219 (2014).
8.Owejan,J.P.,Trabold,T.A.,andMench,M.M.,“Oxygentransport
resistance correlated to liquid water saturation in the gas diffusion